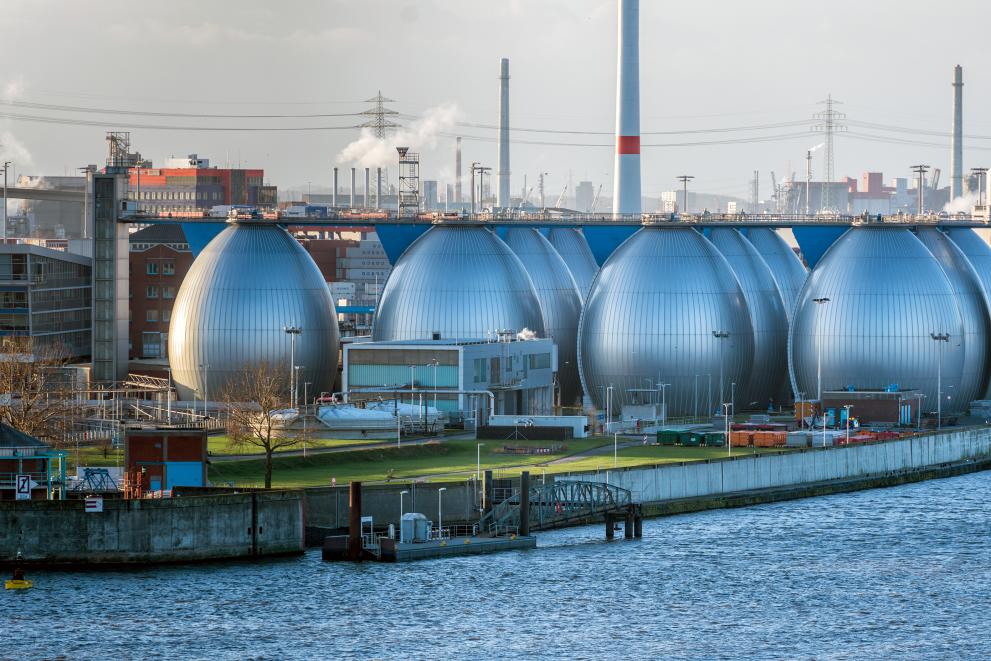
Desalination is a rapidly growing sector of the Blue Economy, with over 2 300 seawater desalination units in the EU, out of over 18 000 worldwide. The Mediterranean is home to most of them. As a result, the EU generates around 9.2 million cubic metres of desalinated water every day (10% of global capacity).
Despite this, a quarter of the world's population lives in countries with chronic water scarcity, and it's predicted that many regions in the EU will face severe water scarcity by 2050 when water demand is expected to increase by up to 30%.
As a result, 3.5 billion people will be at risk of water scarcity by 2025, and the global water deficit is estimated to reach 40% by 2030. However, more freshwater can be made available for domestic, industrial, and agricultural use through the desalination of seawater.
The global population increase and the rise in demand for consumable water have driven the growth of the desalination sector in the past two decades. The global desalination market was valued at EUR 17.4 billion in 2021.
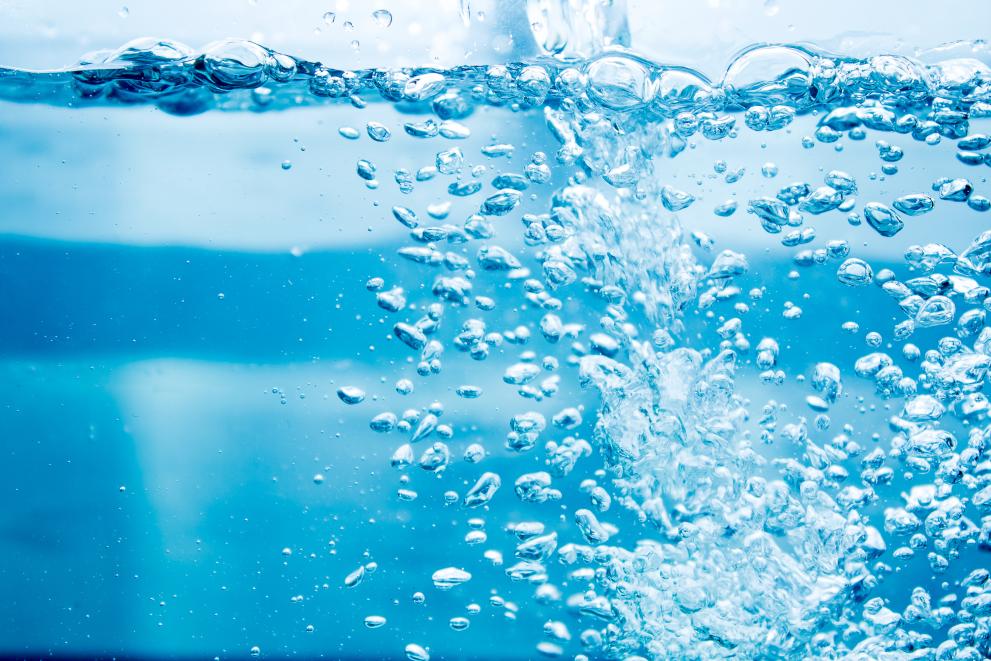
Desalination technology is currently being used to alleviate water shortages in regions with limited freshwater resources, such as large coastal cities, islands, and offshore industrial sites where seawater cannot be used due to its high salinity.
Desalination capacity in Europe has grown significantly in recent decades. However, between 2000 and 2009, Europe's desalination capacity increased to 4.58 million m3/day, with a total investment of EUR 4 billion in engineering, procurement, and construction. Between 2010 and 2019, just 0.84 million m3/day of new capacity was added, at an investment of EUR 630 million. Most additional capacity constructed since 2010 has been in small and medium-sized plants.
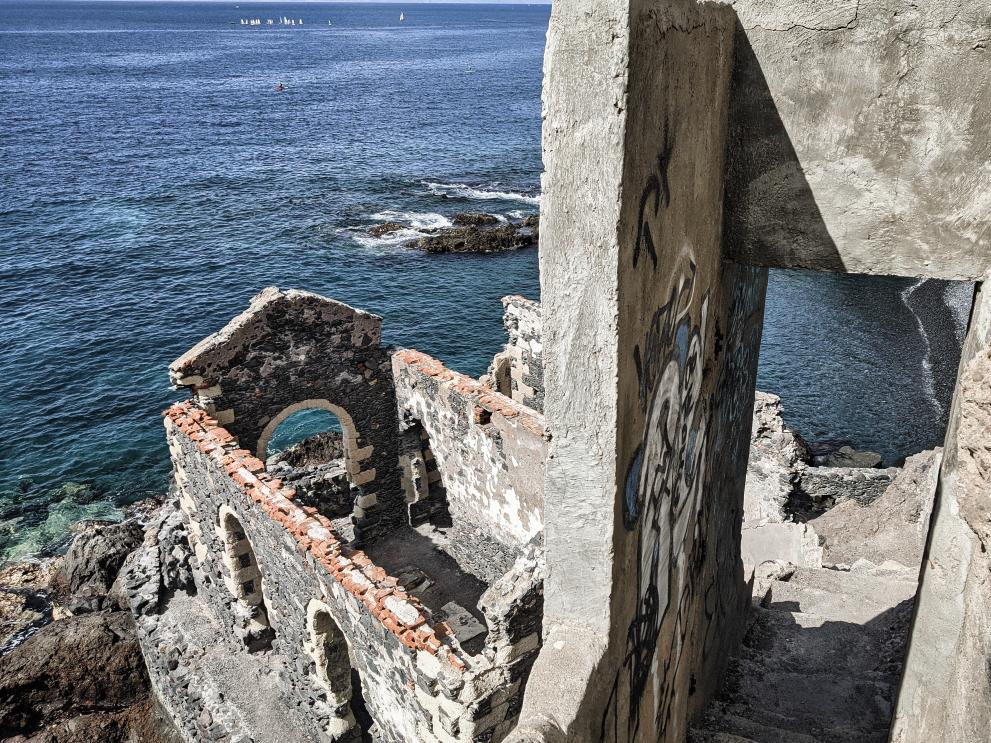
Around 65% of the EU's operational plants are located on or near the coast. Inland plants are also used to produce drinking water and industrial water through a saline/brackish water purification process. In the long run, desalination and other water management options (such as water reuse) are predicted to lessen the impact of climate change on freshwater supply.
The COVID-19 pandemic has hampered the sector’s growth, and manufacturing delays have led to temporary interruptions in a number of construction sites. Production activity has partly resumed in 2021, but the industry is expected to experience a sluggish growth in the near future.
With desalination plants having a lifespan of 20 to 25 years, it is projected that further expenditures would be required in the coming years to modernise or replace obsolete facilities. However, the limited capacity commissioned in Europe over the last decade (2010-2019) demonstrates that this sector of the EU Blue Economy has grown at a slower rate within the EU than it has outside the EU.
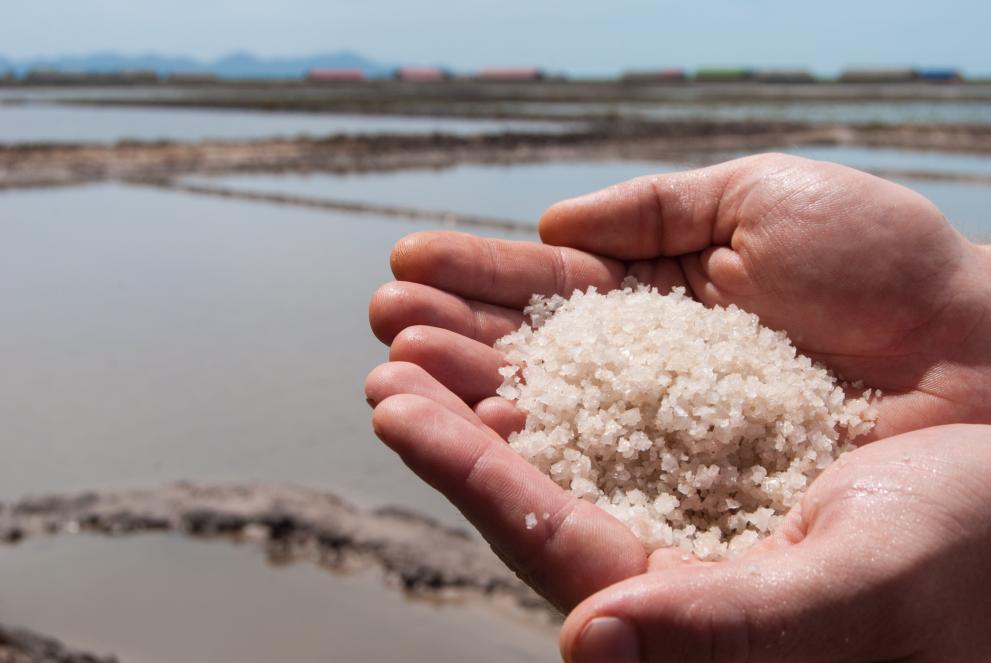
Meanwhile, the Middle East, North Africa, East Asia, the Pacific, and North America have combined global desalination capacity of 78% global capacity.
According to DesalData, Spain has 65% of the EU's desalination capacity and 5.7% of global desalination capacity, with the rest concentrated in Italy (7.5%), France (3.5%), Cyprus (3.4%), Malta (2.9%), and Greece (2.9%). Desalination plants in Northern European nations such as Germany (4%), the Netherlands (3.8%), Belgium (1.9%), and Ireland (1.1%) are primarily used for the production of drinking water and industrial water. Most of the large plants established between 2000 and 2010 served coastal cities in Spain.
Most of the EU's desalination capacity (63%, 5.7 million m3/day) is devoted to the generation of water for municipal public water supplies. Only 3% of the desalination capacity is used to produce drinking water for tourism facilities. The remaining desalination capacity (23%) is used for industrial purposes and irrigation (12% ).